1.1 Introduction
Research Next started with one question:
If we were to develop the university research enterprise from scratch, what would it look like?
This question arises from the growing complexity and interconnectedness of modern research universities. Rather than being insulated places where scientists in white jackets sit in their labs, universities contribute thought leadership in framing and addressing complex challenges; they create jobs and enable economic development; they are integral partners to government, industry, non-governmental organizations, and community engagement ecosystems; they are epicenters of innovation clusters.
Other questions immediately follow from this one, including:
- What are the global megatrends that will influence the future state of the university research ecosystem?
- What are the key problems that research universities must be prepared to address? As an institute historically focused on engineering and technology, how does the Georgia Institute of Technology (Georgia Tech) address techno-social challenges such as sustainability, global security, and equity?
- What research agenda and partnerships will have broad impact?
- How should research ecosystems organize themselves to best enable a group of creative people to do great things? How do we best create and support links between research, education, economic development, and research support?
The underlying premise of these questions — service and interconnectedness — is reflected in the Institute’s core values. Georgia Tech’s strategic plan, released in 2020 [GIT 2020] emphasizes that “Students are our top priority,” recognizing that universities are educators first and foremost. The mission is to make a positive impact on people’s lives and improve the human condition, by educating students, developing leaders, creating knowledge, and advancing technologies. Universities are but one actor in the broader implementation of solutions to societal challenges, and so we must become deeply integrated as trusted partners within the broader ecosystem of governments, companies, and local communities. We must serve as physical “anchor tenants” in innovation clusters where students, researchers, startups, companies, venture capital, and community engagement organizations collaborate and engage.
The objective of this report is to frame these key questions — not to provide prescriptive solutions, but rather to identify the internal and external forces and factors that shape the research ecosystem. This chapter sets the stage by examining the societal engagement dimensions of research universities, the U.S. research university ecosystem, funding mechanisms, and innovation models. Subsequent chapters look at questions around internal organization, external drivers, research content, and partnerships.
IN THIS CHAPTER
1.1 Introduction
1.2 Broader Societal Engagement
1.3 Research University Taxonomy
1.4 Financial Environment
1.5 Research and Development Classifications and Innovation Models
1.6 Organization
1.2 Broader Societal Engagement
The infusion of expertise into broader societal conversations has never been more necessary. The collective challenges we face involve the complex interplay of policy, politics, finance, human behavior, historical context, and technology. Moreover, knowledge and science are easily politicized, and knowledge communities within research universities engage society in a multitude of ways.
As universities work toward a mission of meeting grand challenges and positively affecting humanity, it is important to recognize their cultural attributes — entrepreneurial, competitive, meritocratic, and decentralized — and how they intersect with other organizational cultures in the wider sphere of community advocates, corporations, and government.
Research universities are a subset of a larger group of expert communities, and careful consideration must be given to how they use their expertise as a resource for facilitating complex decisions. Pielke [Pielke 2007] has developed a useful framework, identifying four poles of engagement: pure scientist, issue advocate, science arbiter, and honest broker.
This framework is constructed around two questions shaping research university engagement:
- Is there high or low societal consensus around fundamental values? One example of an issue with high values consensus is the desire to minimize loss of life associated with a hurricane landfall, while low values consensus include such issues as fetal stem cell research or nuclear weapons.
- What is the uncertainty — either technical or political — around a decision? To illustrate, consider the role of expert knowledge in providing input to policy responses to the following low and high uncertainty events: an imminent hurricane landfall and a catastrophic meteorite impact that could occur sometime in the next 100 years.
With issues of high values consensus and low uncertainty, a particular set of knowledge clarifies what the next steps should be to address the issue. Increased knowledge or reduced uncertainty also reduces decision or political uncertainty — for example, a better calculation of the hurricane landfall location improves decision making around evacuations or contingency planning. Experts who are disengaged from the political/policy process can provide this helpful knowledge. Pielke identifies “pure scientists” as those who do their discovery and development divorced from the broader society. They develop knowledge but do not engage with decision makers or those seeking to apply its results. The “science arbiter” seeks to engage society by serving as an expert resource — not explicitly advocating for a given policy decision, but making themselves available to answer specific expert questions.
On the other hand, engagement of expert knowledge is quite different around issues with significant societal disagreement on values or where there is high uncertainty. In these cases, improved expert knowledge often does not clarify the path that rational people should take on a complex topic. As noted by Sarewitz [Pielke 2007a], in a broad range of areas such as climate change, endangered species, air pollution, or biotechnology, the growth of scientific knowledge has not reduced political controversy.
The “issue advocate” and “honest broker” both engage with broader society around decision making. The issue advocate explicitly advocates for policies, supported by their credentials as experts. On the other hand, the honest broker engages in societal discourse but does not advocate for a given policy decision. Even so, the honest broker does deeply integrate within this process and work with other stakeholders to understand the larger policy landscape. For example, in a policy discussion of regulating power plant emissions, the honest broker does seek to understand the interactions of their subject expertise with such issues as jobs, the cost of energy, energy equity, and so forth, all with the goal of expanding the scope of policy options. For the honest broker, the role of experts is to “clarify the implications of their knowledge for action, and to provide such implications in the form of policy alternatives to decision-makers who can then decide among different possible courses of action.” [Pielke 2007]
Pielke also defines a fifth category, the “stealth issue advocate,” as those who present themselves as pure scientists or science arbiters but who are actually advocates for a particular position, seeking to leverage the legitimacy associated with their expertise — for example, an expert who approaches a problem with low societal values consensus and casts it in terms of one with high values consensus.
It is helpful to understand what role research universities are playing as they interject their expertise into wider social, political, and economic discussions. Today, one can easily find examples where universities and experts play any of these roles in different settings, depending upon the issue and the university culture. Moreover, because many of today’s most critical issues are in areas with lower values consensus, there is an opportunity to integrate the honest broker role into the culture of the university. Or, as Pielke writes, “In situations of gridlock, policymakers frequently need new options, and not more science.”
1.3 Research University Taxonomy
Research universities differ widely in subject expertise, size, and mission — and in terms of public versus private; volume of research activity; range of degrees and specializations, including science, technology, arts and humanities, law, and medicine; integration of applied and service functions such as field stations, hospitals, and applied R&D organizations; and degree of admission selectivity. Each of these attributes has a significant influence on the range of activities performed by the universities, their partners, the rules and laws they must work within, the students they educate, and the types of research they conduct.
Given these different categories, it is helpful to understand where Georgia Tech resides within the full group of postsecondary institutions [Indiana 2018].
- Doctoral degree granting institutions represent approximately 10% of all postsecondary degree granting institutions (418 out of 4,324 in 2018) and enroll about 36% of all students in these institutions.
- Of these, 266 are classified as having “very high” or “high” research activity, defined as awarding at least 20 research or scholarship doctoral degrees, with at least $5 million in total research expenditures.
- Of the 266 doctoral universities at very high or high research activity, 74 have “comprehensive” programs without a medical or veterinary school. For the Carnegie classification, “comprehensive” denotes institutions that award research doctoral degrees in the humanities, social sciences, and STEM fields. They may also offer master's or professional degrees in fields other than medicine, dentistry, or veterinary medicine.
- Of these 74 schools, 25 institutions are classified as public and considered more selective based on ACT categorization, as shown in Figure 1.1. That equates to 0.6% of the starting total.
Further, of the 10 institutions of higher education that are institutes of technology or polytechnic institutes and classified as very high research activity (including Carnegie Mellon University and Case Western Reserve University), only three are public universities at the state level: Georgia Tech, Virginia Tech, and the New Jersey Institute of Technology.
Figure 1.1
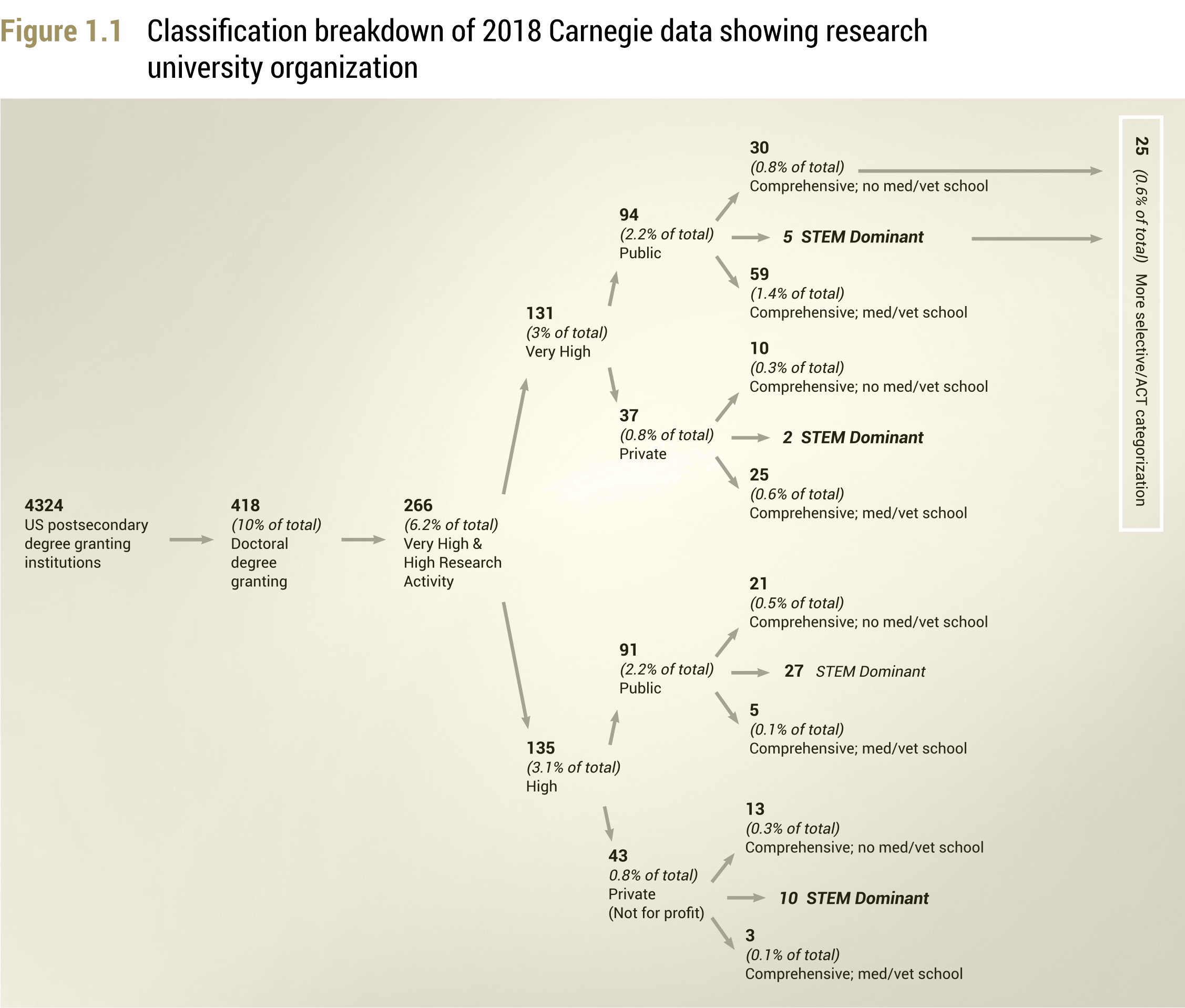
1.4 Financial Environment
Financial support is one of the key enablers of research. Where and how is research funded in the United States? Where do research universities derive their support? Major categories of funding sources for research and development (R&D), as defined by the National Center for Science and Engineering Statistics (NCSES), are: federal government, state and local government, business, higher education, and other nonprofit organizations. Table 1.1 is a summary of NCSES data that shows all R&D expenditures in the U.S. by these five major funding sources from 2011 to 2018.
Table 1.1 U.S. R&D Expenditures, by source of funds: 2011 - 2018
(Millions of current dollars)
Fiscal Year |
All Expenditures |
Federal
|
State & Local
|
Business |
Higher
|
Nonprofit
|
2011 | 426,160 | 127,014 | 4,386 | 266,422 | 13,103 | 15,235 |
2012 | 433,619 | 123,837 | 4,158 | 275,718 | 14,300 | 15,607 |
2013 | 453,966 | 120,130 | 4,244 | 297,168 | 15,378 | 17,046 |
2014 | 475,425 | 118,365 | 4,214 | 318,383 | 16,210 | 18,254 |
2015 | 493,684 | 119,524 | 4,267 | 333,208 | 17,299 | 19,386 |
2016 | 515,641 | 116,492 | 4,481 | 355,545 | 18,484 | 20,640 |
2017 | 547,886 | 120,961 | 4,582 | 381,137 | 19,723 | 21,482 |
2018 | 579,985 | 127,246 | 4,726 | 404,231 | 21,120 | 22,662 |
Source: National Center for Science and Engineering Statistics, National Patterns of R&D Resources (annual series)
As shown in Table 1.1, business has been the single largest source for R&D expenditures over this time period, and it has grown significantly. The business sector’s R&D expenditure in 2018 was 1.5 times of that in 2011. In contrast, the federal government, while the second largest source, has remained flat, actually decreasing from 2011 to 2016. The other sectors have seen stable and gradually increasing R&D expenditures.
University research and development has been supported by all five major funding sources. The R&D expenditures in universities between 2011 and 2018 by source of funding are shown in Table 1.2 below.
Table 1.2 U.S. Higher Education R&D Expenditures, by source of funds: 2011 - 2018
(Millions of current dollars)
|
ALL R&D EXPENDITURES |
FEDERAL |
STATE/
|
BUSINESS |
HIGHER ED |
OTHER NONPROFIT |
|||||
Year |
Total |
Higher Ed.Total |
Total |
Higher Ed. |
Total |
Higher Ed. |
Total |
Higher Ed. |
Higher Ed. |
Total |
Higher Ed. |
2011 | 426,160 | 60,089 | 127,014 | 35,742 | 4,386 | 3,615 | 266,422 | 2,999 | 13,103 | 15,235 | 4,630 |
2012 | 433,620 | 60,086 | 123,837 | 34,967 | 4,158 | 3,560 | 275,718 | 3,170 | 14,300 | 15,607 | 4,899 |
2013 | 453,966 | 61,549 | 120,130 | 33,839 | 4,244 | 3,643 | 297,168 | 3,376 | 15,387 | 17,046 | 5,313 |
2014 | 475,426 | 62,350 | 118,365 | 33,122 | 4,214 | 3,714 | 318,383 | 3,602 | 16,210 | 18,254 | 5,702 |
2015 | 493,684 | 64,624 | 119,524 | 33,555 | 4,267 | 3,773 | 333,208 | 3,843 | 17,299 | 19,386 | 6,154 |
2016 | 515,642 | 67,801 | 116,492 | 34,674 | 4,481 | 3,919 | 355,545 | 4,042 | 18,484 | 20,640 | 6,682 |
2017 | 547,885 | 71,252 | 120,961 | 36,034 | 4,582 | 4,064 | 381,137 | 4,276 | 19,723 | 21,482 | 7,173 |
2018 | 579,985 | 74,722 | 127,246 | 37,202 | 4,726 | 4,159 | 404,231 | 4,551 | 21,120 | 22,662 | 7,690 |
The federal government has been the largest funding source for university research. The second largest source has been the universities’ funds, while other nonprofit organizations, business, and state and local government have been distant runners-up. Business funded 6% of all R&D expenditures in universities in 2018, but that amount only accounted for 1.1% of total business R&D expenditures.
Federal funding of university research has a rich history. A framework for government support of university research was envisaged by Vannevar Bush in his report, Science — The Endless Frontier. It was produced at the close of World War II in response to President Franklin D. Roosevelt’s request for recommendations on how wartime scientific research and technological gains and innovations can be “profitably employed in times of peace” for the “improvement of the national health, the creation of new enterprises bringing new jobs, and the betterment of the national standard of living.”
This framework engendered a new responsibility of the federal government: technology and innovation. It also engendered a new government-university partnership supporting scientific research in universities. Within five years, a number of major federal agencies were established, including the Office of Naval Research (ONR) in 1946 and the National Science Foundation (NSF) in 1950. In addition, the National Institutes of Health (NIH) was significantly expanded and reformulated. ONR, NSF, and NIH soon became the mainstays of federal support for university-based scientific research. Today, support for research and development is a permanent focus of the federal government.
Federal agencies supporting university research include the Department of Defense (DoD), Department of Energy (DOE), Department of Health and Human Services (HHS), National Aeronautics and Space Administration (NASA), National Science Foundation (NSF), and Department of Agriculture (USDA). In 2018, the top three federal agencies with the largest R&D expenditures at universities were HHS, DoD, and NSF, with shares of total federal R&D funding at 55%, 14%, and 13% respectively [NCSES 2018].
The history of corporate funding for university research predates federal funding. In recent decades, particularly since the Bayh-Dole Act in 1980, the share of industry funding of university research has increased gradually and reached a steady rate of about 7% [NAP] in the last decade. As shown in Tables 1.1 and 1.2, industry funding in 2018 amounted to 6% while total industry R&D expenditures enjoyed a share of 69% of all R&D expenditures in the U.S. Although industry funding of university R&D is a relatively small portion, it has been an important component in spurring knowledge-intensive activities in collaborations between academia and industry that have contributed to rapid advancement in industrial innovation and economic development in many fields.
A recent study [Atkinson 2018] found that strong industry funding of a state’s university research pays off in economic benefits to the state, and that positive correlations exist between the share of a state’s university research supported by industry and its strength in key innovation variables such as high-tech startups, venture capital, high-tech jobs, and scientists and engineers. Industry funding is also associated with stronger university technology output. Another study, on the experiences and outcomes of graduate students in STEM fields [Schneider 2007] found that students who collaborated and interacted with industry were nearly five times more likely to produce intellectual property than those who did not.
Finally, nonprofit organizations play an important role in supporting university research and development, spurring innovation, and addressing societal challenges. As shown in Table 1.2, nonprofit organizations sourced 10.3% of the total R&D expenditures in universities in 2018.
1.5 Research and Development Classifications and Innovation Models
University research covers a broad spectrum of disciplines and R&D, from knowledge discovery through systems development. Research and development activities can be classified into three categories [NSF 2018]: basic research, applied research, and experimental development, which includes advanced technology development and systems development. R&D activities must satisfy five criteria: being novel, creative, uncertain, systematic, and transferable and/or reproducible. Another widely used classification is defined by DoD Financial Management Regulation, which classifies R&D into six budget activities (BA): BA1 (code 6.1) - basic research, BA2 (code 6.2) applied research, BA3 (code 6.3) - advanced technology development, BA4 (code 6.4) - advanced component development and prototyping, BA5 (code 6.5) - system development and demonstration, and BA6 (code 6.6) -research, development, test, and evaluation (RDT&E) management support. DoD BA1 and BA2 correspond directly to NSF’s basic research and applied research classifications, respectively. DoD BAs 3-6 correspond to the NSF experimental development classification. A detailed description of DoD’s RDT&E taxonomy and its mapping to the NSF taxonomy can be found in Sargent [Sargent 2020].
Universities have been the primary R&D performing organizations conducting basic research with major funding from federal agencies. Bush’s Science — The Endless Frontier was a formative influence. Indeed, the Office of Scientific Research and Development that Bush led during World War II had succeeded in bringing scientific knowledge to wartime applications. Breakthroughs in basic science drove the development of products to benefit society, and funding research came to be viewed as the surest path to innovation. It espoused a “linear model,” which started with basic research, followed by applied research, and then experimental development to reach production and commercialization. The model, also known as “technology push,” would soon be augmented with another linear model that used product/capability needs as the source of ideas driving R&D and innovation. This model is sometimes referred to as “market pull.”
The ensuing decades witnessed a continuous evolution of innovation processes and models. In the late 1960s and early 1970s, a number of wide-ranging and systematic studies of successful innovation emerged, including Myers and Marquis [Myers 1969], which was sponsored by the NSF “to provide empirical knowledge about the factors which stimulate or advance the application in the civilian economy of scientific and technological findings,” and Rothwell [Rothwell 1994] (and the citations therein). These studies indicated that technology push and market pull models were extreme examples of innovation, and that the more general innovation process was characterized by interactions, which led to the interactive model with feedback loops.
In the 1990s, an innovation process characterized by integration and parallel development was introduced by the Japanese automobile industry for rapid and efficient new product development. U.S. federal R&D agencies and industry quickly adopted this integrated innovation model. For example, ONR has expanded its mission from sponsoring basic research to vertically integrated basic research, applied research, and advanced technology development. A number of successful technological innovations for national security and civilian benefits by ONR and other agencies have been attributed to their use of the integrated innovation model.
As models of innovation evolve, the government’s policy for R&D has evolved and so has the role of university research, the partnerships between government, industry, and university research, as well as the R&D partnership between universities and industry. Increasingly, universities are engaged in research in all R&D categories and have formed close partnerships with industry, which, via knowledge and collaborations, have contributed to the rapid growth of our technology-based economy. As invention, knowledge transfer, and innovation continue to generate new and improved products and technologies, universities will continue to play an integral part in the evolving innovation process. They will continue to be the engine of knowledge creation, while partnering with government and industry in knowledge transfer and innovation.
1.6 Organization
Research Next is organized into four main sections:
“The World Beyond” analyzes the external global factors that are beyond the control of individual research universities, but which affect how they function. The discussion centers around four areas: values, challenges, innovation, and policy.
“Research That Matters” identifies the grand challenges, topics, and methods for conducting research at technology focused research universities that are likely to emerge between now and 2050.
“Working With Others” focuses on partnerships. It considers the range of partners and approaches toward mutually beneficial collaborations, with particular attention on identifying and leveraging the potentially different missions of different partners.
Finally, “The World Within” examines the explicit and implicit structures and functions by which the research ecosystem carries out its mission. How is the work actually done? How can research organizations tackle complex societal challenges and create new research directions; empower and support all researchers; combine research, technology transfer, entrepreneurship, corporate engagement, and economic development; and ensure compliance, research security, and research integrity?
< A Letter from the EVPR Chapter 2: The World Beyond >